
Fiscal 2005
(1) Molecular Level Pursuit of a Spontaneous Cell Death Process by Time-resolved Raman Mapping
It is known that a particle called dancing body (DB) occasionally appears and moves vigorously in a vacuole of a budding yeast (S. cerevisiae) cell. We found that once a DB was formed, the vacuole was eventually lost and the cell died subsequently. This spontaneous death process of a budding yeast cell was studied at the molecular level by time-resolved Raman mapping experiments (Fig. 1). Between 0 min and 5h 50 min, the cell was in the normal state. The "Raman spectroscopic signature of life" at 1602 cm-1 showed high metabolic activity in mitochondria, and phosphlipids (1440 cm-1) and proteins (1002 cm-1) were localized in the outside of the vacuole. At 6 h, a dancing body suddenly appeared and, at the same time, the "Raman spectroscopic signature of life" became very weak, indicating the concomitant lowering of the metabolic activity. At 8 hr 41 min, the metabolic activity entirely stopped but the distribution of phospholipids and proteins still remained unchanged. At 9 hr and 31 min and later, the vacuole was lost and the molecular distribution became random, indicating that the cell was not living any more. In this way, the spontaneous cell death process following the DB formation was traced successfully by time-resolved Raman mapping. This result is of great interest regarding the definition of cell death at the molecular level.
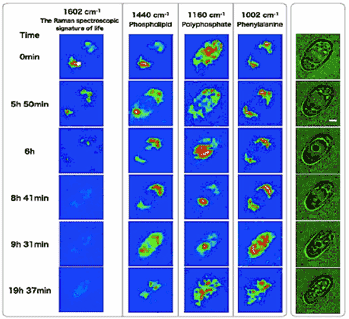
Fig. 1 Time-resolved microscopic images (right, green) and Raman mapping images of a budding yeast cell.
(2) Ultrafast Association/Dissociation Dynamics of Magnesium Sulfate in Water
Our understanding of thermal reactions is much less advanced compared with photochemical reactions. For photochemical reactions, various ultrafast time-resolved spectroscopies are available and they provide detailed information on their reaction mechanism. This is not the case with thermal reactions, which can not be triggered by light. As an alternative to the time domain approach using time-resolved spectroscooies, we have been developing a frequency domain approach based on vibrational band shape analysis. We derived a formula from the Kubo-Anderson theory that describes the band shapes under the asymmetric limit of the two frequency exchange model. According to this formula, the vibrational band shape is a Lorentzian with a peak shift Δω and a band width increase ΔΓ, which are the functions of the exchange rates between the two frequencies. From the concentration dependence of the totally symmetric SO4 stretch band of magnesium sulfate in water (Fig. 2), we were able to determine the association/dissociation dynamics as shown in Fig. 3.
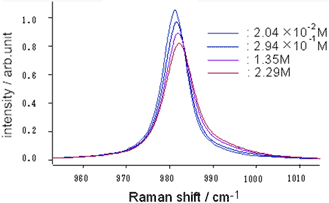
Fig. 2 Concentration dependence of the SO4 symmetric stretch Raman band of magnesium sulfate in water.
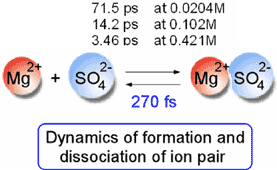
Fig. 3 Association/dissociation dynamics of magnesium sulfate in water.
Refereed Journals
- Molecular-Level Investigation of the Structure, Transformation, and Bioactivity of Single Living Fission YeastCells by Time- and Space-Resolved Raman Spectroscopy. Yu-San Huang, Takeshi Karashima, Masayuki Yamamoto and Hiro-o Hamaguchi, Biochemistry 44, 10009-10019 (2005).
- Vibrational and electronic infrared absorption spectra of benzophenone in the lowest excited tripletstate. Sohshi Yabumoto, Shin Sato and Hiro-o Hamaguchi, Chem. Phys. Lett., 416, 100-103 (2005).
- Photoinduced Processes of Solid Aromatic Compounds by Mid-IR Free Electron Laser. Kensuke Tono, Hiroshi Kondoh, Yasuhiro Hamada, Takahiro Suzuki, Kotatsu Bito, Toshiaki Ohta, Shin Sato, Hiro-oHamaguchi, Akira Iwata and Haruo Kuroda, Jpn. J. Appl.Phys. 44, 10 7561-7567 (2005).
- In vivo time-resolved Raman imaging of a spontaneous death process of a single budding yeast cell. Yasuaki Naito, Akio Toh-e and Hiro-o Hamaguchi, J. Raman Spectrosc., 36, 837-839 (2005).
- Solvent-dependent intra- and intermolecular vibrational energy transfer of W(CO)6 probed with sub-picosecond time-resolved infrared spectroscopy. Motohiro Banno, Shin Sato, Koichi Iwata and Hiro-o Hamaguchi, Chem. Phys. Lett., 412, 464-469 (2005).
- Ion association dynamics in aqueous solutions of sulfate salts as studied by Raman band shape analysis. DaisukeWatanabe and Hiro-o Hamaguchi, J. Chem. Phys. 123, 34508 (2005).
- Ultrabroadband (>2500 cm-1) multiplex coherent anti-Stokes Raman scattering microspectroscopy using a supercontinuum generated from a photonic crystal fiber. Hideaki Kano and Hiro-o Hamaguchi, Appl. Phys .Lett. 86, 121113 (2005).
- Vibrationally resonant imaging of a single living cell by supercontinuum-based multiplex coherent anti-Stokes Raman Scattering microspectroscopy. Hideaki Kano and Hiro-o Hamaguchi, Optics Express 13, 1322-1327 (2005).
- Structure of the S1 state of diphenylacetylene as studied by time-resolved CARS and infrared spectroscopy. Tomonori Nomoto, Taka-aki Ishibashi, Hiromi Okamoto and Hiro-o Hamaguchi, J. Mol. Struct. 735-73,197-202 (2005).
- Cascading third-order Raman process and local structure formation in binary liquid mixtures of benzene and n-hexane. Shinsuke Shigeto, Hideaki Kano, and Hiro-o Hamaguchi, J. Chem. Phys., 122, 064504 (2005).
- 1064 nm near-infrared multichannel Raman spectroscopy of fresh human lung tissues. Youn-Kun Min,Tatsuya Yamamoto, Ehiichi Kohda, Toshiaki ITo and Hiro-o Hamaguchi, J. Raman Spectrosc., 36, 73-76(2005).
- Near-infrared coherent anti-Stokes Raman scattering microscopy using supercontinuum generated from a photonic ctystal fiber. Hideaki Kano and Hiro-o Hamaguchi, Appl. Phys. B, 80, 243-246 (2005).